Use of Narrow Lanes and Narrow Shoulders on Freeways: A Primer on Experiences, Current Practice, and Implementation Considerations
Chapter 2. Performance Based Practical Design and the Role of Operations
Performance Based Practical Design
Performance-Based Practical Design (PBPD) modifies
the traditional "top down, standards first" approach to
a "design up" approach where designers and decision
makers exercise engineering judgment to build up
the roadway and operational improvements from
existing conditions to meet both project and system
objectives. PBPD uses appropriate analysis tools —
such as those discussed in Chapter 4 — to evaluate the
performance impacts of planning and design decisions
in relation to the cost of providing various geometric
elements and operational features.
PBPD should not be viewed as a stand-alone set of
activities. Rather, it is an integral part of a broader
process known as "Performance-Based Planning and
Programming." The Federal Highway Administration
(FHWA) publication "Performance Based Planning and
Programming Guidebook" (Reference 25) describes
the application of performance management
principles within the planning and programming
processes of transportation agencies and regional
entities (e.g., Metropolitan Planning Organization
(MPOs)) to achieve desired performance outcomes for
the multimodal transportation system. Figure 2 shows
the Performance-Based Planning and Programming
(PBPP) process, indicating where PBPD concepts and
activities may be applied. As shown in Figure 2, PBPDrelated
activities can be applied to the preliminary
engineering and design activities, with any cost
savings going to support additional projects as part of
the regional programming process.
PBPD concepts can also be used during planning
activities to help identify strategies and analyze
alternatives.
Figure 3 identifies and summarizes the various
PBPD concepts and potential activities, starting with
"baseline conditions" including design policies and
guidelines, current and projected issues and needs,
and stakeholder concerns; and then moving into
analysis such as developing alternatives, analyzing
these alternatives in terms of improved performance
and costs, coupled with trade-offs and engineering
judgment. The results of these PBPD-related activities
and concepts (i.e., "Moving Forward") is the selection
of the optimal concepts and strategies for design,
the identification of any design exceptions, and the
documentation of the decisions. The optimal design
concepts, along with any associated cost savings, are
fed back into the PBPP framework.
As collectively shown in Figures 2 and 3, with PBPD
designers apply a "design up" approach by using
existing conditions as the baseline and engineer
solutions that meet the project purpose based
on explicitly defined transportation performance
needs as derived from system and regional goals
and objectives. This approach differs from a more
conventional approach of setting project design
criteria based solely on values listed in design
specifications or standards for a set of given
conditions. Designers then evaluate the solutions
against the tradeoffs based on an objective analysis of
performance data. Some of the tradeoffs considered
include the estimated costs for each potential solution,
coupled with due consideration of agency polices,
legal requirements, stakeholder sensitivities, and any
other potential constraints.
Note: Performance-Based Practical Design shown in orange.
Figure 2. Diagram. Framework for Performance-Based Planning and Programming.
(Source: Adapted from Federal Highway Administration document number HEP-13-041, Reference 25)
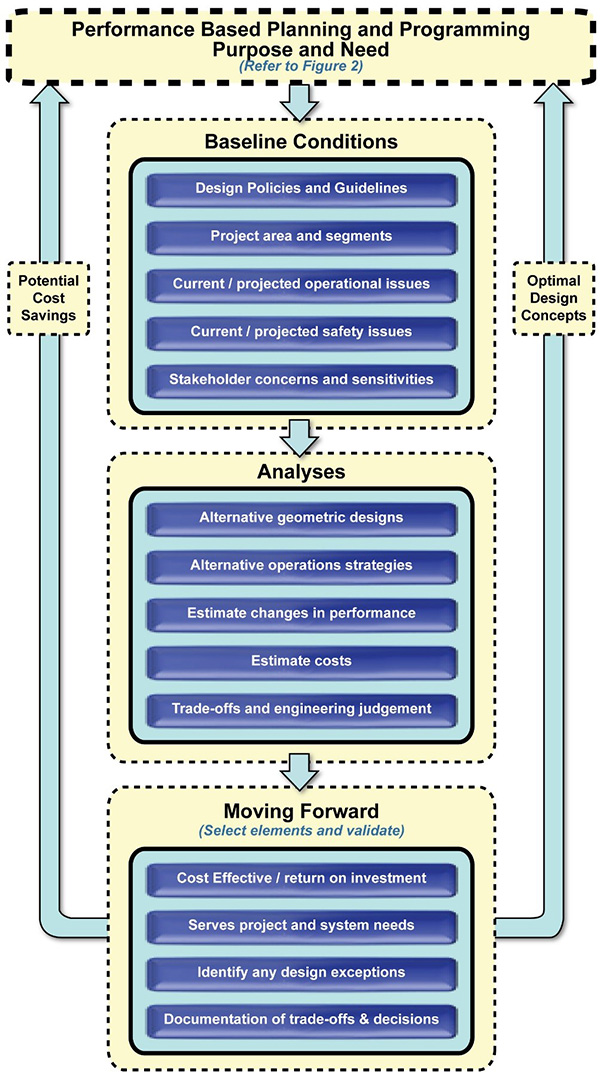
Figure 3. Diagram. Concepts and Activities Associated with Performance-Based Practical Design.
(Figure is based on several Federal Highway Administration documents and presentations on the subject of Performance-Based Practical Design)
A basic tenet of PBPD involves making project
decisions that directly serve performance needs
while considering whether the same investment of
money would yield a greater return on investment
if applied to other system needs and/or priorities.
It is important to document the design decisions
and present them to decision makers showing the
benefits relative to the no-build option. These PBPD
design and decision-making analyses can easily be
transferred to design exception forms for review,
approval and record-keeping.
By implementing a PBPD approach, agencies
may reduce or eliminate project elements that are
determined to be non-essential, resulting in lower
cost and improved value by taking advantage of
existing design flexibility. Agencies may also use the
associated cost saving to deliver a greater number of
projects that yield a greater performance return on
investment than otherwise possible under existing
project development and design approaches.
Relationship Between PBPD and Context Sensitive Solutions
Context-sensitive solutions (CSS) seek a
transportation solution that addresses the needs of
all road users and the functions of the facility within
the context of its setting, considering land use,
users, the environment, and other factors. CSS is a
collaborative, interdisciplinary approach that includes
the viewpoints of all stakeholders in the development
of a shared vision of project goals, and uses a
defined decision-making process. CSS and PBPD
rely on flexibility to achieve results that meet the
project purpose and need. PBPD compliments CSS
by providing performance information that supports
decision-making.
Design Criteria and Design Exceptions
As previously noted, PBPD moves away from the
more conventional "top down, standards first"
approach to more of a performance and valuebased
"design up" approach. Designers that focus
on the relationships between design dimensions and
performance may become less obligated to meet
one or more of the design guidelines, such as those
found in the AASHTO Green Book ("A Policy on
Geometric Design of Highways and Streets"). Design
criteria and standards offer many benefits, including
promoting consistency, establishing a design "norm",
and promoting efficiency in design development.
However, "standard" does not necessarily mean
"best", nor are standards intended to be a substitute
for engineering judgment and context-specific
considerations. PBPD provides planners and
designers with the flexibility to make the optimum
design decisions.
A design exception is a documented decision to
design a highway element or a segment of highway
to design criteria that do not meet minimum
values or ranges established for that highway or
project. Federal regulations (Reference 13) state
that "Approval …may be given on a project basis
to designs which do not conform to the minimum
criteria as set forth in the standards, policies, and
standard specifications." A design exception is NOT
an indication of failure or a "flawed" design; rather
it is a necessary and legitimate process to allow
professional and engineering judgment in the design
process, providing a useful "tool" for employing
practicality and flexibility in design decisions in a
design-up approach such as PBPD.
As noted in the FHWA document “Mitigation
Strategies for Design Exceptions” (Reference 14)
there are a broad range of reasons why design
exceptions may be considered and found to be
necessary. Some of these include the following:
- Impacts to the natural environment
- Social or right-of-way impacts
- Preservation of historic or cultural resources
- Sensitivity to context and community values
- Construction or right-of-way costs
Widening the roadway footprint, including the
possibility of additional right-of-way, will certainly
impact the last bullet, and may impact one or more
of the other bullets. Adding a lane by narrowing the
existing lanes within the existing roadway footprint
can help reduce or even eliminate the concerns
noted above in the bulleted list that so often apply to
projects that end up widening the roadway.
A final notice published in the Federal Register on
May 5, 2016 completed FHWA's effort to update
the policy regarding controlling criteria for design,
applicable to projects on the National Highway
System. FHWA reduced the number of controlling
criteria from 13 to 10 for Interstate highways, other
freeways, and roadways with design speed ≥ 50 mph,
and now applies only 2 of those criteria to low speed
roadways (non-freeways with design speed < 50
mph). FHWA also clarified when design exceptions
are needed and the documentation that is expected
to support such requests.
FHWA has adopted new policies to modify highway
design standards that encourage greater flexibility in
order to achieve a design that best suits the desires
of the community, while satisfying the purpose for the
project and needs of its users. As an example, FHWA
published revisions to current federal policy that will
help reduce cost and speed up the design of local
roads and streets. In 1985, thirteen design criteria
were prioritized because of their perceived impact
on operations and safety. Under the new policy, ten
criteria will be prioritized for high speed roadways,
and only two criteria will be emphasized for lowerspeed
roads such as rural roads that become main
streets through smaller towns and cities. This will
provide state and local engineers to develop flexible
design solutions that meet local travel needs and
goals.
Chapter 8 of the Green Book recommends shoulder
widths for freeways shown in Table 4. Additionally,
the AASHTO policy on design standards for the
Interstate highway system requires a 10 ft. paved
right (outside) shoulder. Shoulder widths less than
the values shown in Table 4 will also require a design
exception under the existing and proposed FHWA
policy on Controlling Criteria.
Moving the inside travel lane closer to the roadway
edge — including part time use of the shoulder as a
travel lane — may also impact the horizontal alignment
(to be renamed as "horizontal curve radius" under
the proposed FHWA policy on Controlling Criteria)
and sight distance as shown in Figure 4, and may
therefore also require a design exception.
Controlling Criteria for Design Exceptions, 2016
- Design Speed*
- Lane Width
- Shoulder Width
- Horizontal Curve Radius
- Superelevation
- Maximum Grade
- Stopping Sight Distance
- Cross Slope
- Vertical Clearance
- Design Loading Structural Capacity*
*Design Speed and Design Loading Structural Capacity apply to all roads on the National Highway System
Table 4. Recommended Shoulder Widths for Freeways.
Side of Roadway |
DDHV for truck traffic (veh/hr) |
Total numbers of freeway lanes |
Recommended shoulder width (ft) |
Right Shoulder |
≤ 250 |
All |
10 |
Right Shoulder |
> 250 |
All |
12 |
Left Shoulder |
≤ 250 |
Less than 6 |
4 |
Left Shoulder |
≤ 250 |
6 or more |
10 |
Left Shoulder |
> 250 |
All |
12 |
(Source: NCHRP Report 783: Evaluation of the 13 Controlling Criteria for Geometric Design; adapted from Chapter 8 of the AASHTO Green Book)
Figure 4. Diagram. Potential Impact on Sight Distance From Moving the Left-Most Travel Lane To the Inside.
Transportation Systems Management and Operations
Transportation Systems Management and
Operations (TSMO) — also often referred to simply as
"operations" — is defined as:
"Integrated strategies to optimize the performance
of existing infrastructure through the implementation
of multimodal and intermodal, cross-jurisdictional
systems, services, and projects designed to preserve
capacity and improve security, safety, and reliability of
the transportation system."
Intelligent Transportation System (ITS) technologies
— be they devices for monitoring traffic flow on the
roadways, hardware and software at Transportation
Management Centers (TMCs), and/or "Connected
Vehicle" applications — are crucial to the success
of these operations strategies. ITS represents the
“enabling technology for operations.”
TSMO strategies — coupled with the supporting ITS
technology — are a most important aspect of delivering
transportation services to customers. Experience
has shown that aggressive applications of these
operations strategies can, in effect, "take back" much
of the capacity lost due to congestion and disruptions.
Operations strategies also enhance safety, promote
reduced emissions, and increase system reliability.
Perhaps most importantly, actively managing the
transportation network can improve travelers'
experiences, providing them with real-time information
and choices throughout the trip chain — from origin
to destination — leading to network performance
optimization and increased efficiency. TSMO
strategies are relatively low cost (compared with
adding capacity), much quicker to implement (two to
three years), and offer substantial benefits (with very
positive benefit-cost ratios).
FHWA recommends an "objectives-driven,
performance-based approach" for including
"operational and management strategies to improve
the performance of existing transportation facilities"
in the planning process. This objectives-driven,
performance-based approach to planning for
operations within a metropolitan area — conducted
in collaboration among planners, transportation
providers, operators, and other stakeholders — is shown
in Figure 5.
The activities shown in Figure 5 parallel the PBPP and
PBPD concepts identified in previous Figures 2 and
3, including the development of potential strategies
based on goals, objectives, and needs; and then
evaluating and subsequently selecting strategies
in terms of performance and cost. Moreover, lowcost,
rapidly deployable, and flexible treatments — as
provided by many TSMO strategies — all fall under
the collective umbrella of PBPP and PBPD. Table 5
provides a list of TSMO strategies that may be used
in conjunction with narrow lanes and/or shoulders as
part of the PBPD process.
Figure 5. Flowchart. An Objectives-Driven, Performance-Based Approach.
(Adapted from Federal Highway Administration, Reference 25)
Table 5: Transportation System Management and Operations Strategies Typically Used On Freeways and the Potential Relationships to Performance-Based Practical Design.
TSMO Strategy |
Description |
Potential Use and Value in Narrow Lanes/Shoulders |
Incident Management
|
The systematic, planned, and
coordinated use of human,
institutional, electrical, mechanical,
and technical resources to reduce
the duration and impact of
incidents, and improve the safety
of motorists, crash victims, and
incident responders.
|
Used to address safety and
reliability concerns of narrow lanes
or the loss of the shoulder as a
vehicle refuge.
|
Ramp Management
|
The application of control
devices, such as traffic signals,
signing, and gates to regulate the
number of vehicles entering or
leaving the freeway, or to smooth
out the rate at which vehicles enter
and exit the freeway.
|
Metering the traffic that enters the
freeway from on-ramps can help
prevent flow breakdown on the
mainline, and improve safety in
merge and weaving areas that may
be impacted by the use of narrow
lanes and/or shoulders.
|
Managed Lanes (HOV,HOT)
|
Highway facilities or a set of lanes
where operational strategies
are proactively implemented and
actively managed to optimize traffic
flow and vehicular and person
throughput. These strategies
typically involve pricing, vehicle
eligibility, and access control.
|
Used to improve travel time and
reliability for vehicles carrying the
most passengers or those willing
to pay an additional fee for using
the lane. The use of narrow lanes
and shoulders may provide the
opportunity to add or expand such
lanes.
|
Traveler Information (511, apps on Smartphones, DMS)
|
A combination of strategies for
enabling better traveler decision
making throughout the trip chain —
before, during, and near the end of
a trip.
|
Allows drivers to adjust their
route, time of travel, or mode, thus
lessening demand on key facilities
at peak times. DMS can also alert
motorists that queues, significant
slowdowns, or blocked lanes are
ahead — as may result from narrow
lanes or shoulders — thus reducing
rear-end crashes and improving safety.
|
Dynamic Speed Limits
|
Adjusts speed limit (or advisory)
displays based on real-time traffic,
roadway, and/or weather conditions.
They can be applied to an entire
roadway segment or individual
lanes. This "smoothing" process
helps minimize the differences
between the lowest and highest
vehicle speeds.
|
Used to reduce speeds in advance of
congestion, or perhaps in advance
of segments with narrow lanes,
limited shoulder widths, or reduced
sight distance (e.g., requiring a
reduced design speed as part of
the design exception process). Is
often used in conjunction with part
time shoulder use, dynamic lane
assignment. (Refer to Figure 6)
|
Dynamic Lane Assignment
|
Dynamically closing or opening
individual traffic lanes as warranted
and providing advance warning of
the closure(s), typically through lane
control signs, to safely merge traffic
into adjoining lanes.
|
Used to open and close a part time
lane (e.g., part time shoulder use),
or to close lane(s) upstream of a
crash or disabled vehicle (e.g., with
no shoulder for refuge). One or
more lanes may also be closed to
allow emergency vehicles to reach
the crash scene quicker, particularly
if there are narrow shoulders. This
strategy is often used in conjunction
with dynamic speed limits.
|
Dynamic Junction Control
|
Dynamically allocating lane access
on mainline and ramp lanes in
interchange areas. This may consist
of assigning lanes dynamically either
for through movements, shared
through-exit movements, or exitonly.
|
Used to better allocate available
capacity at interchange areas and
reduce the amount of weaving and
merging. Is typically used with some
sort of dynamic lane assignment.
May be deployed to identify if the
ramp shoulder is open to traffic and
which mainline lanes can access
the ramp; or may be deployed to
promote safe merging operations
during use of the mainline shoulder.
|
Incorporating the consideration of TSMO strategies
into the PBPD concepts and activities, designers and
decision makers can expand the variety of options
available to them, including perhaps the ability
to postpone or reduce the need for conventional
capacity improvements. Additionally, TSMO
strategies may also help mitigate some of the safety
and reliability impacts of PBPD solutions that result
in less than full standard geometric design decisions,
thereby providing solutions and support for any
design exceptions. For example, dynamic speed limits
and dynamic lane assignment strategies may be used
in this context as shown in Figure 6.
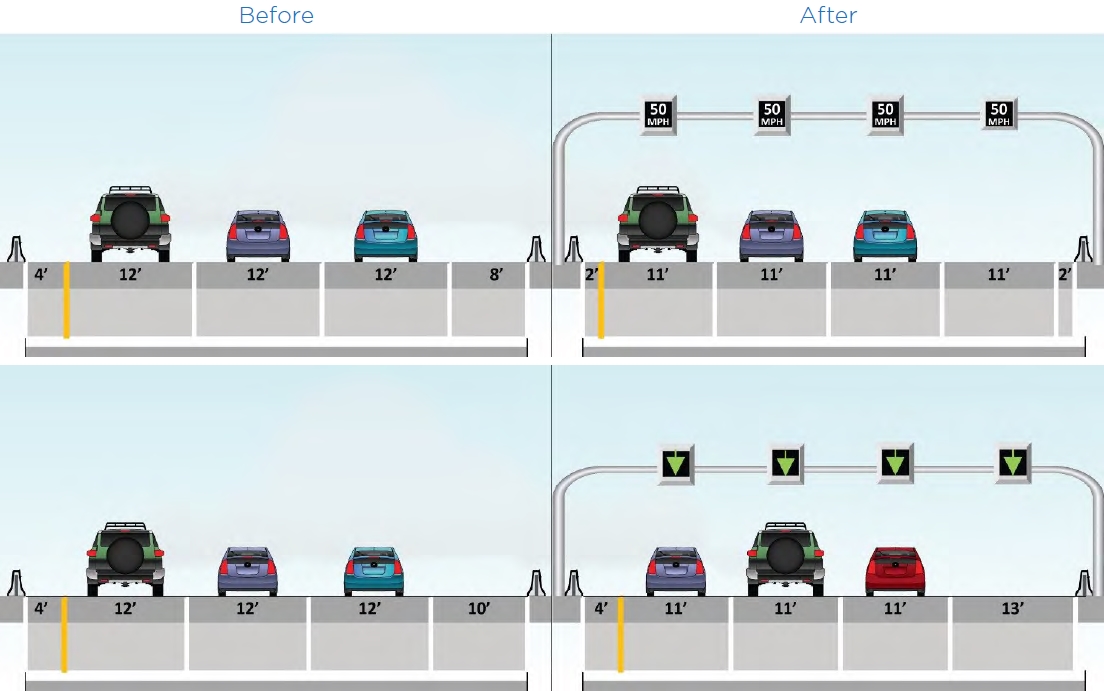
Figure 6. Diagram. Example of Dynamic Speed Limits and Dynamic Lane Assessment in Support of Narrow Lanes and Shoulders.
In summary, PBPD and TSMO — along with Context
Sensitive Solutions and value engineering — are very
complimentary as shown in Figure 7. Their respective
approaches have much in common, and they all
strive for the same goal — namely, providing a wellperforming
transportation system using the most
cost-effective improvements.
The FHWA website for PBPD (Reference 12) identifies
notable attributes for PBPD as listed below. The
phrase "and TSMO" can be added immediately after
"PBPD" in this list and still ring very true.
- PBPD (and TSMO) focuses on performance improvements that benefit both project and system needs.
- Agencies make sound decisions based upon performance analysis.
- By scrutinizing each element of a project's scope relative to value, need, and urgency, a PBPD (and TSMO) approach seeks a greater return on infrastructure investments.
- PBPD (and TSMO) strengthens the emphasis on planning-level corridor or system performance needs and objectives when planning, scoping and developing individual projects.
- PBPD (and TSMO) can be implemented within the Federal-aid Highway Program regulatory environment utilizing existing flexibility. PBPD does not eliminate, modify, or compromise existing design standards or regulatory requirements.
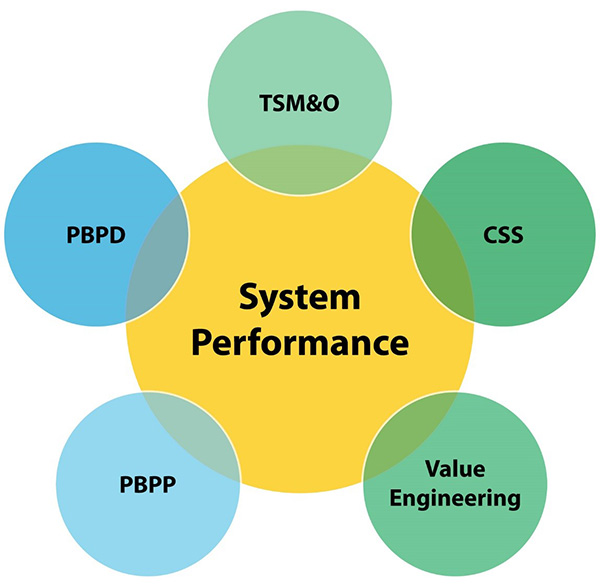
Figure 7. Diagram. Overlapping Relationship between Performance-Based Practical Design, Performance-Based Planning and Programming, Transportation System Management and Operations, Context SensitiveSolutions, and Value Engineering.