Traffic Analysis Toolbox Volume XII:
Work Zone Traffic Analysis – Applications and Decision Framework
Chapter 7. Evaluating Factors for MOTAA Recommendations
This chapter presents a mix of quantitative and qualitative factors that should be considered in a Maintenance of Traffic Alternatives Analysis (MOTAA), in addition to the mobility-based performance measures generated through the traffic modeling analysis. The first section of this chapter describes the various factors and details how they can be incorporated into the evaluation and comparison of alternatives. The second section is dedicated to measures of safety in relation to a work zone traffic analysis. Typical work zone safety analysis utilizes historical crash data to measure the safety impacts of the work zone, as well as to identify potential mitigation measures that minimize safety impacts. This section also describes an additional form of measuring the safety impacts of projects through surrogate measures of safety, and how to generate the safety measures, and where these considerations fit into the MOTAA process.
7.1 Work Zone Key Factors
While modeling analysis tools produce certain measures that could be used to evaluate and compare work zone alternatives, there are additional qualitative and quantitative measures that may not be easily captured by such tools. This section describes how these key factors can be used to support the development and evaluation of work zone alternatives.
This section is structured to present the key factors into two categories: 1) development of potential work zone alternatives; and 2) evaluating and comparing work zone alternatives. Subfactors within the first category include those related to traffic operations, public information and awareness, and construction and contracting options. Subfactors within the category of evaluating work zone alternatives include environmental and land use impacts, project financial factors, and road user costs.
Development of Potential Work Zone Alternatives
There are several factors that an agency may need to consider early on in the project development process in order to develop the potential work zone alternatives that will be modeled or evaluated during the alternatives analysis. These factors have the ability to shape the development of the alternative. Additionally, these factors also can address which supporting strategies could be incorporated within those alternatives. Incorporated into the work zone alternatives, these strategies have the potential to affect the mobility and safety impacts and performance measures of various alternatives. Three such factors, i.e., traffic operations, construction and contracting factors, and public information and awareness, are discussed in this section.
Traffic Operations
The Traffic Operations factor includes strategies and programs that serve to improve and/or optimize mobility and safety within the work zone area. This factor is considered in order to evaluate and compare work zone alternatives and to assess the effectiveness of certain mitigation measures that could help the agency meet their project goals and objectives. There are certain strategies that support the key factor of traffic operations. The following lists examples of such strategies as well as how they can be used to shape and support work zone alternatives.
- TDM Strategies – Demand management strategies provide mode shift incentives that encourage people to use alternative modes of transportation as a way of relieving traffic congestion. TDM strategies can include transit, HOV, and park-and-ride incentives and programs. These strategies should be considered as mitigation strategies that can support the work zone alternatives and aid an agency with meeting their goals and objectives. For instance, an agency may choose to implement park-and-ride and transit incentives in order to reduce congestion during peak travel in the work zone project area.
- Traffic Operational Improvements – Traffic operational strategies include projects and programs that help optimize or improve traffic flow along transportation facilities. Some examples of these improvements include signal phasing/coordination, geometric improvements, and temporary signals. Similar to TDM, traffic operational improvements can support existing work zone alternatives in order to minimize or mitigate the work zone’s mobility impacts. For instance, one potential work zone alternative may be to establish diversion routes. To ensure these detour options are able to handle the additional demand, signal phasing/coordination improvements can optimize traffic flow and operations along these routes.
- Incident and Enforcement Strategies – Incident and enforcement strategies can aid in clearing incidents and/or diverting traffic quickly and efficiently. These strategies could include improving service patrols response, traveler information, and signage. Such strategies can help support work zone alternatives and aid the agency in achieving certain project goals and objectives, especially those related to improving safety and reducing mobility impacts in and around the project area.
- Work Zone Safety – Increasing work zone safety strategies can aid in preventing incidents along work zone corridors. Work zone safety strategies can serve as forms of mitigation techniques to achieve certain safety goals and objectives. Additionally, alternatives could be evaluated for how effectively they address work zone safety project goals and objectives such as reducing worker incidence and crash frequency and severity rates. Safety is discussed in greater detail in Section 8.2.
Construction and Contracting Factors
The construction and contracting factors address the various options that may have the ability to optimize the project schedule, construction duration, use of resources, and costs. Consideration of such factors can aid an agency in shaping their list of work zone alternatives and in achieving project goals and objectives. Various strategies that may be considered as part of these factors can serve as alternatives or may be incorporated into the work zone alternatives considered for the MOTAA. Example strategies related to construction and contracting include the following:
- Construction Phasing/Staging Strategies – Staging of projects refer to how the contractor will position the equipment and material. Phasing refers to the sequencing of various aspects of a project. These types of strategies can serve as potential work zone alternatives that address different options for work zone strategies (i.e., full road closure, lane closures, and detours) and phasing options for when, and in which order, construction tasks and/or project sections are completed.
- Contracting Strategies and Project Delivery Methods – These types of strategies provide alternative ways of contracting the work and delivering the project. These could be incorporated into certain work zone alternatives as ways of accelerating construction duration and they may be considered as options for work zone alternatives analyzed within an MOTAA. Some examples of alternative contracting strategies that have the potential to optimize and/or accelerate project delivery and schedule include:
- A+B Bidding – This contract combines the cost to perform the work (component A) and the time to complete the project (component B). The bidder with the lowest overall A and B combined bid is awarded the contract.
- Incentive/Disincentive Contracts – With these contracts, the contractor is provided a monetary incentive for completing the project ahead of schedule and is penalized with a disincentive fee for delays. A similar version of the incentive/disincentive contract is the “No excuse incentives” contract where a contractor is given a firm completion date with incentives for completing the work by or before that date.
- Design-Build – This is a project delivery method where the agency combines procurement for both the design and construction services into a single contract from one private entity.
- Construction Manager/General Contractor – This is a two-phase project delivery method in which a construction manager is selected for both the preconstruction and construction services of a project.
- Other Innovative Construction Strategies – These include innovations in construction strategies and use of materials that aid in improving the project schedule. Examples include using precast members, prefabricated structural systems, rapid strength concrete, and polymer modified concrete. These strategies also could be incorporated into the development of particular work zone alternatives considered for an MOTAA.
Public Information and Awareness
The public information and awareness factor include strategies that inform nearby residents, businesses, and facility users of the project’s progress, updates, and impacts. Informing all relevant stakeholders that may be involved or impacted by the project is key for project approval, keeping on schedule, and reducing mobility and safety impacts in and around the project area. This factor and supporting strategies should be considered within an MOTAA to support work zone alternatives and mitigate impacts of the project. Strategies that fall into this factor category may include:
- Media Outlets for Project Background and Information – These types of public information and awareness strategies provide the community background on the project, goals, and objectives as well as a forum for the public to voice comments, concerns, and suggestions. Such strategies could include community and stakeholder meetings, the distribution of brochures and press releases, creation of a promotional web site, and provision of a hotline to provide information and updates regarding the project and construction progress. These strategies inform the agency of specific concerns and issues that may impact the effectiveness and/or success of the project and work zone alternatives considered. Furthermore, such strategies can support particular work zone alternatives. For instance, public information strategies could inform users of detour routes and when to avoid the project area due to lane and road closures.
- Traveler Information – Traveler information strategies are another form of public information techniques that can help an agency support work zone alternatives and achieve their project goals and objectives. Traveler information strategies include web sites providing vital traveler information (i.e., 511), highway advisory radio (HAR), and dynamic message signs (DMS). These provide road users pre- and en-route information regarding expected travel times, congestion levels, and detour options due to the construction. Such strategies can be incorporated into work zone alternatives to help mitigate safety and mobility impacts.
Evaluating and Comparing Work Zone Alternatives
The previous chapters on alternatives analysis presented some of the types of performance measures that could be extracted from some of the modeling and analysis tools. These outputs primarily consist of mobility measures that could be used to compare and evaluate alternatives. As previously mentioned, there are additional qualitative and quantitative factors that also should be considered in an alternatives analysis. This section presents several types of factors that should be incorporated into the criteria and measures used to select a preferred work zone alternative. These factors, as discussed in the following sections, include environmental and land use impacts, project costs, and road user costs (RUC).
Environmental and Land Use Impacts
Environmental and land use factors require the agency to evaluate how a work zone alternative may impact the environment, as well as the residential and business community near the project area. Consideration for these factors can be used to ensure that the potential work zone alternatives are able to meet certain environmental standards. Additionally, these should be incorporated into an alternatives analysis or decision-making criteria in order to minimize negative impacts on surrounding residents, businesses, and services. Such considerations can include the following:
- Right-of-Way – An agency should consider any need for the acquisition or purchase of right-of-way for the specific work zone alternatives involved. The amount of right-of-way should be incorporated into evaluating the land use and community impacts of the work zone alternatives.
- Air Quality Impacts – This factor accounts for the vehicle emissions and greenhouse gas impacts of the project and/or work zone alternative. The air quality impacts of the work zone alternatives can be used as a criterion for evaluating and comparing the work zone alternatives, as well as identifying the need for certain mitigation strategies to reduce such impacts.
- Noise Impacts – This factor accounts for noise-level issues caused by the project that substantially exceed noise abatement criteria or existing noise levels in the area. Considerations for such impacts also can be used to compare and evaluate different work zone alternatives as well as identify mitigation strategies to relieve or reduce noise impacts.
- Impact to Retail Neighborhoods, Businesses, Employment Centers, Major Activity Hubs – Residential and business community impacts should be a factor considered in comparing the impacts of various work zone alternatives. Consideration of this factor can be used to identify certain mitigation measures that can minimize the negative impacts of the project or work zone alternative on the community. Examples may include customer access to facilities and businesses, parking loss, change in property values, and decrease in sales and profits as a result of the work zone.
- Political Sensitivity – This factor considers the risk to the project due to any political issues and/or opposition to the project. The project and proposed alternatives would be evaluated early on in the project to assess the feasibility and probability of it obtaining approval. This factor can be used to eliminate certain alternatives that may potentially generate political opposition or involve unwarranted risk.
Project Costs
This factor includes the costs associated with implementing the project through the particular work zone or construction strategy selected, costs associated with incorporated mitigation and monitoring strategies, and any right-of-way costs. Such costs are typically a major criteria element of whether an agency will consider or recommend a particular work zone alternative. Several work zone-related project costs include:
- Costs for Implementing Work Zone Alternative Strategies – These costs could be related to the costs of pursuing the alternative strategies listed in the Construction Methodology and Innovation section versus the cost of traditional construction and contracting strategies.
- Costs for Mitigation and Monitoring Strategies – These costs would be related to implementing any mitigation measures identified to minimize the impacts of the project. It also should cover cost considerations for any monitoring activities put in place.
- Right-of-Way Costs – These costs are related to the acquisition of right-of-way for the project.
Road User Costs
Another factor for consideration when comparing and evaluating work zone alternatives is road user costs. Work Zone Road User Cost (WZ RUC) is defined as the incremental cost or value incurred by highway users and the community at-large as a result of a work zone alternative. (Work Zone Road User Costs – Concepts and Applications. Federal Highway Administration, U.S. Department of Transportation, FHWA-HOP-12-005, December 2011.) WZ RUC primarily refers to monetary and nonmonetary components of work zone impacts. These could include quantitative measures such as user delay, vehicle operating cost, and crash cost. WZ RUC also includes considerations for qualitative measures such as the environmental, business, and societal impacts of the work zone project. The costs of such impacts can be used to evaluate and compare the different work zone alternatives.
The following lists the typical components and considerations needed for calculating the WZ RUC. A work zone example also is used to illustrate the key points and processes involved for calculating the cost of delay. For the more detailed step-by-step process for calculating WZ RUC, including how the work zone example featured in this document can be used to calculate vehicle operating costs and other RUC components, please refer to the Work Zone Road User Costs – Concepts and Applications. (Work Zone Road User Costs – Concepts and Applications. Federal Highway Administration, U.S. Department of Transportation, FHWA-HOP-12-005, December 2011.)
Work Zone Example Project Description
The following example illustrates the work zone RUC methodology as detailed in the FHWA’s Work Zone Road User Costs: Concepts and Applications. In this example, a pavement rehabilitation work zone project is being performed on a six-lane, urban interstate. The work zone features a single-lane closure in the northbound direction of the interstate. The following illustrates how the methodology can be used to compute the total delay costs generated by the work zone.
Step 1 – Inputs for Demand-Capacity Analysis
One of the first components of the WZ RUC calculation process is the data collection effort. The inputs needed to estimate the work zone impacts for computing the RUC of a project include: hourly traffic demand, traffic composition, work zone capacity, travel speeds, work zone configuration, and the maintenance of traffic (MOT) strategy(s).
For the example, the input data would include the following:
- The northbound lane carries an average daily traffic of 33,000 vehicles, of which eight percent are single-unit trucks and four percent are combination trucks.
- There are also ramp closures. Traffic volumes on the closed ramps equal 1,000 vehicles per day, of which three percent are single-unit trucks and two percent are combination trucks.
- The work zone project area is two miles long with a 24-hour/day single-lane closure. The estimated construction duration period is 20 days.
- The unrestricted upstream approach has a speed limit posting of 55 mph. In the work zone area, the speed limit posted is 45 mph.
- There is a three-mile detour road provided where the average speed is 40 mph.
Step 2 – Computing the Monetary Value of Delay
The total delay time is calculated as the sum of delay due to speed changes, speed reductions, stopping, queue, and detours that occur as a result of the work zone’s implementation. The monetary value of delay due to these components is computed by multiplying the total delay time with the monetary value of travel time. The monetary value of travel time is the sum of the value of personal, business, and truck travel time, as well as the cost of freight inventory delay (for trucks only) and cost of vehicle depreciation (for all vehicles). The following steps describe how to calculate the monetary value of delay using the example.
1. Value of travel time for passenger cars.
- To determine the value of travel time for passenger cars, the analyst must first determine the total delay time. The total delay time is the sum of speed change delay, reduced speed delay, stopping delay, queue delay, and detour delay. Figure 38 presents how the total delay time of 10,192.6 vehicle-hours per day is computed for the example work zone.
- The next step is to determine travel delay costs per passenger by multiplying the total delay time with the unit travel time values per passenger.
- To determine these travel time values the analyst must estimate the unit value of personal and business travel time. For this example, personal travel time is determined to be $20.88/vehicle-hour. Business travel time is valued at $36.89/vehicle-hour.
- The analyst can then compute the hourly time value of passenger cars using the weighted average of travel time values considering both personal and business travel time. 2009 NHS statistics state that 93.7% of passenger cars are expected to be on personal travel while 6.3% are on business. Using these statistics and the hourly time values computed in the previous step, the weighted average travel time values can be computed for passenger cars through the following: Hourly time value of passenger cars = (93.7% * $20.88) plus (6.3%*$36.89) = $21.89/hour.
- To estimate the delay costs for passenger cars on the northbound lanes, the analyst can use the following:
- Percent of passenger cars on NB lanes = 88%
- Estimated delay time for all vehicles = 10,192.6 vehicle-hours/day
- Estimated delay time for passenger cars = 0.88* 10,192.6 = 8969.49 vehicle-hours/day
- Estimated delay costs for passenger cars = 8969.49*$21.89/hour = $196,342.10 per day
Value of travel time for trucks.
- To determine the travel delay costs for trucks, the analyst must determine the truck travel time value. For this example, the hourly time value of single-unit trucks is $23.06/hour. The hourly time value of combination trucks is $29.65/hour.
- The analyst will then have to estimate the delay costs for both classes of trucks for the project using percentage of trucks, delay time for all vehicles, and the travel time unit values determined in the previous step.
- For single-unit trucks:
- Percent of single-unit trucks in example = 8%
- Estimated delay time for all vehicles = 10,192.6 vehicle-hours/day
- Estimated delay time for single-unit trucks = 8%*10,192.6 = 815.4 vehicle-hours/day
- Estimated delay costs for single-unit trucks = 815.4 * $23.06/hour = $18,803.31/day
- For combination trucks:
- Percent of combination trucks in example = 4%
- Estimated delay time for combination trucks = 4%*10,192.6 = 407.7 vehicle-hours/day
- Estimated delay costs for combination trucks = 407.7*$29.65 = $12,088.42/day
- Estimated delay for all trucks = $18,803.31 plus $12,088.42 = $30,891.73
3. Time-related vehicle depreciation costs for all vehicles.
The third step in determining total delay costs for all vehicles is to determine the time-related vehicle depreciation costs for the three vehicle types: passenger cars, single-unit trucks, and combination trucks.
- Passenger Cars – Through previous calculations, the analyst has determined that the percent of passenger cars is 88 percent and that the estimated delay time for this vehicle class is 8,969.49 vehicle-hours/day. Therefore, if the hourly time-related depreciation cost for passenger cars is $1.225/hour, the estimated depreciation cost for passenger cars = 8969,49 * $1.225/hr or $10,987.62/day.
- Single-Unit Trucks – Previous calculations have shown that this example can expect 8 percent of all vehicles to be single-unit trucks. Additionally, this percentage of trucks generates a total delay time of 815.4 vehicle-hours/day. If the hourly depreciation cost for this truck class is $3.09/hour, the estimated depreciation cost for single-unit trucks is = 815.4 * $3.09/hr= $2,519.59/day.
- Combination Trucks – Previous calculations have shown that there are 4 percent combination trucks in this example that generate a total delay time of 407.7 vehicle-hours/day. If the hourly depreciation cost for combination trucks is $9.29/hour, then the estimated time-related depreciation cost for this vehicle class is equal to 407.7 * $9.29/hour = $3,787.53/day.
- The estimated time-related depreciation cost for all vehicles = $10,987.62 plus 2,519.59 plus 3,787.53 = $17,294.74/day.
4. Cost of freight inventory delay.
The final delay value component is the cost of freight inventory delay. This cost is determined by multiplying the number of trucks carrying freight by the hourly cost of freight inventory valuables.
- The first step in this process is to estimate the hourly cost of freight inventory valuables. For single-unit trucks this is $0.18/hour and for combination trucks this is $0.31/hour.
- The next step is to estimate the number of loaded freight trucks. For this example, the number of loaded single-unit trucks is 1,874 and loaded combination trucks are 964.
- The third step is to estimate the cost of freight inventory delay. With the average delay time for a vehicle per day at .309 hour/vehicle/day, the cost of freight inventory delay for trucks can be computed as the product of average payload * number of trucks * average delay time.
- For single-unit trucks = $0.18/hour * 1,874 * 0.30 hour/vehicle/day = $104.23/day
- For combination trucks = $0.31 * 964 * 0.309 = $92.34/day
- Cost of freight inventory delay for all trucks = $104.23 +92.34 = $196.57/day
5. The Total Delay Costs.
The total delay costs is equal to the sum of value of travel time for passenger cars, the value of travel time for trucks, time-related depreciation costs for all vehicles, and cost of freight inventory delay.
Total Delay Costs for the example = $196, 342.10 plus 30,891.73 plus 17,294.74 plus 196.57 = $244,725.10/day.
Figure 38. Work Zone Travel Delay Analysis
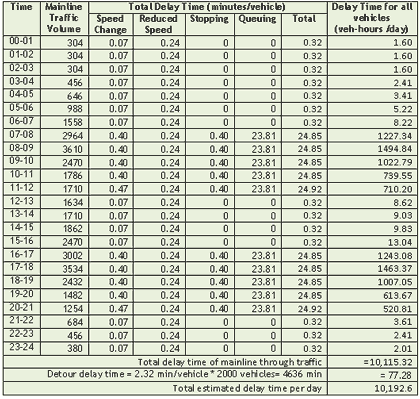
Step 3 – Computing Vehicle Operating Costs (VOC)
Vehicle operating costs (VOC) are the costs incurred by road users as a result of vehicle use. It is calculated as the sum of vehicle operating costs associated with the speed change, stopping, queue idling, and detouring that occur as a result of the work zone. The vehicle operating costs is an aggregate measure of the consumption of the following resources while driving a vehicle between two points. It includes considerations for: fuel consumption, engine oil consumption, tire wear, repair and maintenance, and mileage-related depreciation.
Step 4 – Computing Crash Costs through Crash Analysis
The crash costs associated with work zones are a function of the changes in crash rates due to the presence of the work zone. Crash-related inputs for this part of the WZ RUC analysis include: crash rate at work zones, crash severity rating, and unit cost of crashes.
Step 5 – Emissions Analysis
Work zone-related emission costs are computed by determining the costs associated with the additional vehicle emissions resulting from reduced speeds and queuing. Vehicle emissions considerations include air pollutant emissions and greenhouse gases. Emissions cost is the product of the vehicle miles traveled, emission rate, and unit costs (cost/mile) by emission type. Several models such as the Static Emission Factor Model and Dynamic Instantaneous Emission Model can be used in order to estimate emission rates. The monetary values for emissions are typically derived from economic analyses of health impacts caused by the different types of emissions. Two sources of emission costs include the California Department of Transportation (Caltrans) estimates and HERS-ST Technical Report.
Step 6 – Accounting for Nonmonetary and Qualitative Factors
Additionally, WZ RUC analysis also takes into consideration additional factors such as noise, business, and societal impacts. Further details on these considerations are provided in the previous section on Environmental and Land Use Factors.
I-15 Devore Example
Background and Overview
In 2004, Caltrans applied an innovative, fast-track reconstruction program to a heavily traveled Long-Life Pavement Rehabilitation Strategies (LLPRS) project on I-15 in the area of Devore in southern California. This area covered a 4.5-kilometer (2.8-mile) stretch of badly damaged concrete lanes. It was rebuilt in only two single-roadbed continuous closures (also called “extended closures”) totaling 210 hours, using counterflow traffic (opposite direction to the main traffic flow) and 24-hour-per-day construction operations. Traditional nighttime-only closures would have required 10 months worth of work. Instead, the reconstruction took 19 days, with each extended closure for one roadbed lasting 9 and one-half days.
The following describes the factors project managers and engineers considered before and during construction. Such strategies assisted decision-makers with selecting the optimal and most cost-effective work zone alternatives, use of resources, and traffic operation mitigation strategies to employ.
Development of Work Zone Alternatives and Mitigations Measures
The I-15 Devore Project included four closure work zone alternatives: 72-hour weekday, 55-hour weekend, 1-roadbed continuous (24 hours per day, 7 days per week), and 10-hour nighttime. (Lee, E., and D. Thomas. Accelerated Reconstruction of I-15 Devore Corridor. Public Roads, Volume 70, No. 4, 2007.) The factors, supporting strategies, and mitigation measures that were incorporated into the development of these strategies include the following:
- Operational Considerations – The project’s traffic operational strategies included the use of moveable barriers during construction, which provided dynamic lane configurations that helped to balance traffic and accommodate peak direction traffic. The travel demand management strategies implemented with this project included free commuter bus service to promote ridesharing. The enforcement and monitoring strategies included the provision of a project command center (agency coordination and monitored traffic and construction using CCTVs), the Construction Zone Enhanced Enforcement Program, which improved traffic control and enforcement in the construction work zone, and increased funding for California Highway Patrol to increase enforcement within the project area.
- Construction Method and Innovation – The project used innovative materials such as rapid-strength concrete mix, incentive/disincentive contracts.
- For pavement design and material alternatives, Caltrans used a software model to make comparisons from the perspective of production scheduling and traffic inconvenience. As a result, Caltrans selected: 1) rapid-strength concrete with a 12-hour curing time rather than fast-setting hydraulic cement concrete with a 4-hour curing time; 2) a 15-centimeter (6-inch) new AC base, rather than a 15-cm lean concrete base; and 3) a widened 4.3-meter (14-foot)-wide lane, rather than the usual 3.7-meter (12-foot)-wide lane tied to a new concrete shoulder on the outermost truck lane.
- Public Information and Awareness – The project included a multifaceted outreach program. The project utilized systems that provided real-time work zone travel information through changeable message signs, the project web site, and a telephone hotline. Caltrans received and shared feedback from the local community through the High Desert Commuter Advisory Committee. Prior to construction, large employers and affected businesses were informed through project fliers, public meetings, and media outreach.
Factors Considered in Alternatives Analysis and Decision-Making
The following factors were considered when evaluating and deciding among the four closure scenarios:
- Project, Road User Costs, Construction Duration, and Mobility Impacts:
- A preconstruction analysis was conducted in order to arrive at the optimal construction closure scenario and pavement design and material alternatives.
- Project engineers used traffic simulation modeling analysis software to arrive at an optimal and economical scenario for rehabilitation closures.
- The analysis compared the scenarios by cost, schedule duration, and delay impacts as shown on Table 77. The analysis concluded that the continuous/extended closure scenario would be the most economical.
- Compared with traditional 10-hour nighttime closures, the preconstruction analysis indicated that the extended closure scenario would generate about 80 percent less total closure time, about 30 percent less road-user cost due to traffic delay, and about 25 percent less agency cost for construction and traffic control.
- The I-15 northbound roadbed was closed for reconstruction, and northbound traffic was switched to the southbound side via median crossovers at the ends of the work zone. Traffic in the two directions shared the southbound lanes as counterflow traffic separated by the moveable barrier. The same process was repeated for reconstruction of the southbound roadbed.
Table 77. Closure Options for I-15 Devore Project
Closure Scenario |
Schedule Comparison |
Traffic Delay |
Cost Comparison
(Million Dollars) |
Number of Closures |
Closures
(Hours) |
Road User Cost
(Million Dollars) |
Maximum Delay (Minutes) |
Agency Cost |
Total Cost to Users and Agency |
72-Hour Weekday |
8 |
512 |
6.6 |
75 |
12.6 |
19.2 |
55-Hour Weekend |
10 |
550 |
12.7 |
196 |
15.1 |
27.8 |
1 Roadbed Continuous |
2 |
400 |
6.1 |
196 |
9.9 |
16.0 |
10-Hour Nighttime |
220 |
2,200 |
10.0 |
36 |
20.4 |
30.4 |
7.2 Work Zone Safety Analysis
This section discusses work zone safety factors and considerations within an MOTAA process, with a focus on surrogate measures of safety as it relates to work zone traffic analysis. The section introduces measures of safety by providing an overview of the differences between traditional traffic safety analysis versus the development of surrogate safety measures as a way of predicting and preventing future crashes. The section then provides examples of surrogate safety measures and their role in an MOTAA procedure.
Purpose of a Work Zone Safety Analysis
In general, most work zone assessment studies agree that crash rates appear to be higher during work zone periods than preconstruction conditions. The diverse and sometimes conflicting range of results from work zone research occur because the various projects studied often incorporate different work zone configurations and strategies, and exhibit different types of roadway and traffic characteristics. For instance, incident rates are typically seen to increase by approximately 20 to 30 percent within work zones relative to the area’s normal crash rates. (Ullman, G.L., M.D. Finley, J.E. Bryden, R. Srinivasan, and F.M. Council. Traffic Safety Evaluation of Nighttime and Daytime Work Zones. NCHRP Report 627, Transportation Research Board, Washington, D.C., 2008.) Additionally, among these work zone incidents the predominant crash type seen are rear-end crashes. (Mahoney, K.M. et al. Design of Construction Work Zones on High-Speed Highways. NCHRP Report 581, Transportation Research Board, Washington, D.C., October 2006.) Due to the significant potential increases in crash frequencies during work zone conditions, it is important for agencies to conduct work zone safety analyses to identify potential mitigation and/or preventative strategies that could improve safety within the project area. Additionally, when conducting a work zone safety analysis, agencies should be aware of the potential variations in results from work zone safety research. When possible, agencies and analysts should conduct work zone safety studies and analyses customized to their specific work zone’s design and strategies, as well as the project area’s traffic and road geometric characteristics.
Traditional Work Zone Safety Analysis
Work zone crashes are typically divided into two categories: crashes due to the presence of the work zone and crashes that would have occurred regardless of the work zone. Traditional work zone safety analysis consists of reviewing existing crash patterns prior to construction. These historical crashes are reviewed in order to estimate the potential crashes that would have occurred regardless of the work zone’s presence. In order to estimate potential crashes due to the work zone, traditional safety analysis typically requires reviewing crash patterns of a previous work zone project that has similar characteristics to the subject project. Although the traditional work zone safety analysis procedures may vary slightly between agencies, the process typically involves the following steps:
- Identify completed work zone projects that share similar characteristics to the new project;
- Review crash data for the similar work zone projects;
- Review existing preconstruction crash data at the project location;
- Determine best practices of design and mitigation measures for the new project based on review conducted in Step 2; and
- Based on results from Steps 3 and 4, determine if there are crash patterns that could be addressed through design or mitigation strategies that minimize crash rates and severity prior to and during construction.
Using historical crash data, accidents also can be valued and used to compare the safety implications of work zone alternatives. For example, a United Kingdom Department for Transport (DfT) sponsored computer program, Quadro (Queues and Delays at Roadworks) estimates the increase in accident rates along a transportation facility as a result of the presence of construction. (The Quadro Manual Part 2: The Valuation of Costs in Quadro. July 2004. Accessed January 11, 2012.) It then converts this increase into a monetary value that can be used to evaluate and compare different work zone alternatives. In order to perform this calculation, the Quadro tool utilizes historical crash data. Crash rates for each facility type are collected during preconstruction conditions and with various types of work zone traffic control strategies. These rates are then multiplied by the estimated traffic volumes in the work zone to estimate the potential number of crashes that will occur when the work zone project is implemented. The difference between the crash rates at preconstruction and during construction is then converted to a monetary value by multiplying the average comprehensive cost for each crash severity level. (Highway Work Zone Analysis. Wisconsin Transportation Economic Analysis Guidelines. Accessed January 11, 2012.)
Table 78 shows the Quadro road user cost outputs from a Wisconsin Department of Transportation work zone traffic analysis of a 24-hour freeway lane closure project. In this example, Wisconsin DOT analyzed the mobility and road user cost impacts associated with one of the project’s diversion routes.
Table 78. Quadro Road User Cost Output Example
 |
Output Result |
Average Accident Numbers (Personal Injury Accidents/Day) |
Main route without works |
0.0767 |
Main route with works |
0.0736 |
Diversion |
0.1385 |
Impacts on Road Users |
Road user costs per day (dollars) |
$45,587 |
Congested hours per day (delays Exceeding 15 Minutes) |
3 |
Overview of Surrogate Safety Measures
While traditional safety analysis primarily focuses on mitigation measures based on historical crash data, surrogate measures of safety addresses predictive and preventative strategies to improve safety within work zones. Surrogate measures of safety are especially helpful in the absence of reliable or accurate historical crash data. The results of surrogate safety measures research and studies can serve a variety of different purposes:
- Such studies can be used to determine how certain project, facility, and driver behavior characteristics impact the probability of accidents occurring;
- These measures can determine how certain project and/or traffic characteristics relate to crash severity; and
- Surrogate safety measures can provide further insight on which mitigation measures will be most effective in minimizing the safety impacts of the work zone.
Research Developments and Analysis of Safety Analysis Surrogate Measures
Several research efforts have analyzed and developed surrogate safety measures with applications for work zones. Surrogate measures of safety can be characterized into two categories: conflict events and driver behavior characteristics. This section presents surrogate safety measures research conducted for these two specific categories.
Surrogate Safety Measures: Conflict Events
Much of the research has dealt with surrogate measures in regards to traffic conflicts. Conflict events can be described as potential crash situations due to safety concerns regarding the roadway design. Conflict events can lead to potential rear-end or angled collisions due to turns, merges, and lane changes. Some examples of surrogate measures regarding traffic conflicts that could be used to evaluate the severity of conflict within work zones include time to collision, post encroachment time, and deceleration rates. (Gettman, D., and L. Head. Surrogate Safety Measures from Traffic Simulation Models. The 82nd TRB Annual Meeting, National Research Council, Washington, D.C., January 2003.)
There also have been development efforts for modeling and analysis tools that provide insight on surrogate measures of safety in relation to conflict analysis. One of these tools is the Surrogate Safety Assessment Model (SSAM), which is an FHWA-sponsored project that analyzes narrowly averted vehicle-to-vehicle collisions in order to assess and predict the safety of a traffic facility and prevent or reduce the frequency of accidents occurring. (Surrogate Safety Assessment Model (SSAM). Publication FHWA-HRT-08-049, Federal Highway Administration, U.S. Department of Transportation, Washington, D.C., May 2008.) SSAM combines microsimulation and automated conflict analysis to calculate the following surrogate safety measures:
- Minimum time-to-collision (TTC);
- Minimum post encroachment time (PET);
- Initial deceleration rate (MaxD);
- Maximum speed (MaxS);
- Maximum speed differential (DeltaS); and
- Vehicle velocity change had the event proceeded to a crash (DeltaV).
SSAM provides outputs that include a table of conflicts identified in the analysis, including information regarding, time, location, vehicle information, and measures of conflict severity. Figure 39 provides a snapshot of the SSAM user interface with conflict icons color coded to display varying values of time-to-collision.
Figure 39. SSAM Screenshot
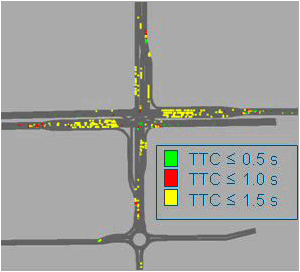
(Source: Federal Highway Administration, 2008.)
Surrogate Safety Measures: Driver Behavior Parameters
Other research efforts study the relationships between surrogate measures of safety and driver behavior characteristics. Several of these studies provide insight on how certain driver behavior characteristics can impact the frequency and severity of crashes. Some examples of how driver behavior characteristics have been used to develop surrogate measures of safety include:
- Evaluating the relationship between driver behavior parameters and rear-end collisions – One study by Sun and Benekohal (2004) looked at driver gap characteristics for evaluating the risk of rear-end collisions for vehicles in platoons. (Sun, D., and R. Benekohal. Analysis of Car Following Characteristics for Estimating Work Zone Safety. The 83rd TRB Annual Meeting, National Research Council, Washington, D.C., Paper No. 04-4883, January 2004.) This research quantifies the relationship between car-following parameters and safety performance measures in work zones. It involves studying the variations of time headway for different car-following models and work zone types.
- Evaluating the relationship between driver behavior parameters and crash severity – A research study by Li and Bai (2008) evaluated the use of measures such as deceleration rate, maximum speeds, and speed differentials at work zones to develop a measure that provides insight on the severity of potential collisions within construction zones. (Li, Y., and Y. Bai. Development of Crash-Severity-Index Models Based on Work Zone Crash Severity Analyses. The 87th TRB Annual Meeting, National Research Council, Washington, D.C., January 2008.)
- Evaluating the safety at ramp junctions – Additionally, certain microsimulation tools can generate outputs and performance measures related to surrogate measures of safety. For instance, AIMSUN can be used for evaluating the safety at ramp junctions using measures such as speed differential, maximum speed of the follower, and the deceleration rate of the follower vehicle during ramp-merging events. (Torday, A., and A. Dumont. Safety Indicator for Microsimulation-Based Assessments. Swiss Federal Institute of Technology, Laboratory of Traffic Facilities, LAVOC-EPFL, Lausanne, Switzerland, 2002.)
Use of Surrogate Safety Measures Within a MOTAA
For the most part, surrogate safety measures have been used at the research or academic level. Currently, there are very few instances of real-world applications of surrogate safety measures, particularly in work zones. Despite this, surrogate measures of safety can still serve a role within the MOTAA process. Some examples of how these measures could be used in evaluating work zone alternatives include the following:
- Surrogate measures of safety can be utilized as criteria elements for evaluating and comparing alternatives during the decision-making stage of an MOTAA.
- Additionally, the surrogate safety measures can be used to identify safety issues regarding the project or work zone alternative design. Such measures can provide insight on how to adapt or modify a particular alternative in order to meet a certain level of safety.
- Alternatively, they can be used to identify and determine the effectiveness of certain mitigation strategies for addressing work zone safety.
Summary of Surrogate Measures of Safety
Table 79 provides a summary of the example surrogate safety measures discussed. The table highlights the analysis method employed, the required data inputs, outputs, and limitations in evaluating and utilizing the safety measure.
Table 79. Summary of Safety Surrogate Measures
Measure |
Analysis Method |
Input Data |
Purpose of Research
and the Measure |
Limitations |
Reference |
Crash Severity Index (CSI) |
Statistical analysis |
Driver Characteristics, Time, Environmental Conditions, Road Conditions, Crash Information, Traffic Control |
CSI models were designed to quantify the risk level of a work zone with a numerical value between zero and one.
A CSI of one indicates a very high-risk level in a given work zone, which infers that a fatal crash might take place if a crash occurs. |
Predicted CSI values for fatal crash cases were not consistent with the actual severity outcomes collected with the research. Further research with crashes of varying severity levels is required to improve the CSI models. |
Li, Y., and Y. Bai, 2008, Development of Crash-Severity-Index Models based on Work Zone Crash Severity Analyses, Transportation Research Board Conference Proceedings. |
Platoon and Gap Analysis |
Statistical analysis |
Work Zone Type, Hourly Volume, Maximum Platoon Size |
The probability of a rear-end crash and how many vehicles might be involved in this crash. |
Work zone safety performance is difficult to evaluate due to the lack of reliable work zone crash data. |
Sun, D., and R. Benekohal, 2004, Analysis of Car-following Characteristics for Estimating Work Zone Safety, Transportation Research Board Conference Proceedings. |
Time-to-Collision (TTC), Post Encroachment Time (PET), and Deceleration Rate (DR) |
SSAM, in conjunction with microsimulation |
Conflict Point, Vehicle Speed, Acceleration, Driver Behavior, Traffic Signalization |
Lower TTC, Lower PET, and Higher DR indicate higher probability of collision. |
Large quantity of input data may be needed specific to the location. |
Gettman, D., and L. Head, 2008, Surrogate Safety Measures from Traffic Simulation Models, Transportation Research Board Conference Proceedings. |
MaxS and DeltaS |
SSAM, in conjunction with microsimulation |
Conflict Point, Vehicle Speed, Acceleration, Driver Behavior, Traffic Signalization |
The measures provided insight regarding the likely severity of the (potential) resulting collision if the conflict event had resulted in a collision instead of a near-miss. Higher MaxS and DeltaS indicate higher severity of the resulting collision. |
Large quantity of input data may be needed specific to the location. |
Gettman, D., and L. Head, 2008. Surrogate Safety Measures from Traffic Simulation Models, Transportation Research Board Conference Proceedings. |
Unsafety Density Parameter (UD) |
Postprocess microsimulation modeling results |
Car-following model parameters, section length, position, speed, and max breaking capacity of follower and lead vehicle, number and duration of simulation steps |
The analysis highlighted the difference in safety level between fluid and “jerked” traffic flow using UD as the safety indicator. The analysis compared a ramp section under a metering scenario and a without-metering scenario. Results showed higher UD values are correlated with higher levels of congestion. |
The analysis has primarily been used for comparing scenarios. Its use also is limited to evaluating highway segments since its focus is primarily on the potential for rear-end collisions. The accuracy of the modeling results also is dependent on the quality of the driver behavior parameters. |
Torday, A., and A. Dumont, 2002, Safety indicator for Microsimulation-Based Assessments, Swiss Federal Institute of Technology, Laboratory of Traffic Facilities, LAVOC-EPFL, Lausanne, Switzerland. |